Fat oxidation process -
The overall equation for the β-oxidation of palmitoyl-CoA 16 carbon atoms is as follows:. Because each shortened fatty acyl-CoA cycles back to the beginning of the pathway, β-oxidation is sometimes referred to as the fatty acid spiral.
The fate of the acetyl-CoA obtained from fatty acid oxidation depends on the needs of an organism. It may enter the citric acid cycle and be oxidized to produce energy, it may be used for the formation of water-soluble derivatives known as ketone bodies, or it may serve as the starting material for the synthesis of fatty acids.
The amount of ATP obtained from fatty acid oxidation depends on the size of the fatty acid being oxidized. For our purposes here. Calculating its energy yield provides a model for determining the ATP yield of all other fatty acids. The breakdown by an organism of 1 mol of palmitic acid requires 1 mol of ATP for activation and forms 8 mol of acetyl-CoA.
Recall from the metabolism of carbohydrates that each mole of acetyl-CoA metabolized by the citric acid cycle yields 12 mol of ATP. The complete degradation of 1 mol of palmitic acid requires the β-oxidation reactions to be repeated seven times.
Thus, 7 mol of NADH and 7 mol of FADH 2 are produced. Reoxidation of these compounds through respiration yields 3 and 2 mol of ATP, respectively.
The energy calculations can be summarized as follows:. However, in terms of grams, there is a big difference between the energy that can be generated per gram of glucose and per gram of fatty acid. In the carbohydrate metabolism module, we determine that the oxidation of 1 mol of glucose produces 38 ATP moles, that is, 38 x 7.
That is the amount of energy produced by 1 mol, or g of glucose. In other words, 1 gram of glucose produces 1. For a fatty acid, such as palmitic acid, we are able to produce ATP molees per mol of palmitic acid, that is, x 7. One mole of palmitic acid equals grams. Therefore, the complete oxidation of palmitic acid produces 3.
The fact that carbons atoms in fatty acids are more reduced than the carbon atoms in glucose explains the difference in the amount of energy produced by their oxidation. In the liver, most of the acetyl-CoA obtained from fatty acid oxidation is oxidized by the citric acid cycle.
However, under certain metabolic conditions such as starvation or diabetes mellitus, the rate of fatty acid oxidation increases to provide energy. This leads to an increase in the concentration of acetyl-CoA.
The increased acetyl-CoA cannot be oxidized by the citric acid cycle because of a decrease in the concentration of oxaloacetate, which is diverted to glucose synthesis.
Therefore, some of the acetyl-CoA is used to synthesize a group of compounds known as ketone bodies : acetoacetate, β-hydroxybutyrate, and acetone. Two acetyl-CoA molecules combine, in a reversal of the final step of β-oxidation, to produce acetoacetyl-CoA.
The acetoacetyl-CoA reacts with another molecule of acetyl-CoA and water to form β-hydroxy-β-methylglutaryl-CoA, which is then cleaved to acetoacetate and acetyl-CoA. Most of the acetoacetate is reduced to β-hydroxybutyrate, while a small amount is decarboxylated to carbon dioxide and acetone.
The acetoacetate and β-hydroxybutyrate synthesized by the liver are released into the blood for use as a metabolic fuel to be converted back to acetyl-CoA by other tissues, particularly the kidney and the heart.
Under normal conditions, the kidneys excrete about 20 mg of ketone bodies each day, and the blood levels are maintained at about 1 mg of ketone bodies per mL of blood. In starvation, diabetes mellitus, and certain other physiological conditions in which cells do not receive sufficient amounts of carbohydrate, the rate of ketone body formation in the liver increases further, to a level much higher than can be used by other tissues.
The excess ketone bodies accumulate in the blood and the urine, a condition referred to as ketosis. When the acetone in the blood reaches the lungs, its volatility causes it to be expelled in the breath.
The sweet smell of acetone, a characteristic of ketosis, is frequently noticed on the breath of severely diabetic patients. Because two of the three kinds of ketone bodies are weak acids, their presence in the blood in excessive amounts overwhelms the blood buffers and causes a marked decrease in blood pH to 6.
This decrease in pH leads to a serious condition known as acidosis. One of the effects of acidosis is a decrease in the ability of hemoglobin to transport oxygen in the blood. In moderate to severe acidosis, breathing becomes labored and very painful. The body also loses fluids and becomes dehydrated as the kidneys attempt to get rid of the acids by eliminating large quantities of water.
The lowered oxygen supply and dehydration lead to depression; even mild acidosis leads to lethargy, loss of appetite, and a generally run-down feeling.
Therefore, when glucose levels are low, triglycerides can be converted into acetyl CoA molecules and used to generate ATP through aerobic respiration. The breakdown of fatty acids, called fatty acid oxidation or beta β -oxidation , begins in the cytoplasm, where fatty acids are converted into fatty acyl CoA molecules.
This fatty acyl CoA combines with carnitine to create a fatty acyl carnitine molecule, which helps to transport the fatty acid across the mitochondrial membrane. Once inside the mitochondrial matrix, the fatty acyl carnitine molecule is converted back into fatty acyl CoA and then into acetyl CoA.
The newly formed acetyl CoA enters the Krebs cycle and is used to produce ATP in the same way as acetyl CoA derived from pyruvate. Figure 3. Click for a larger image. During fatty acid oxidation, triglycerides can be broken down into acetyl CoA molecules and used for energy when glucose levels are low.
If excessive acetyl CoA is created from the oxidation of fatty acids and the Krebs cycle is overloaded and cannot handle it, the acetyl CoA is diverted to create ketone bodies.
These ketone bodies can serve as a fuel source if glucose levels are too low in the body. Ketones serve as fuel in times of prolonged starvation or when patients suffer from uncontrolled diabetes and cannot utilize most of the circulating glucose.
In both cases, fat stores are liberated to generate energy through the Krebs cycle and will generate ketone bodies when too much acetyl CoA accumulates. In this ketone synthesis reaction, excess acetyl CoA is converted into hydroxymethylglutaryl CoA HMG CoA.
HMG CoA is a precursor of cholesterol and is an intermediate that is subsequently converted into β-hydroxybutyrate, the primary ketone body in the blood. Figure 4. Excess acetyl CoA is diverted from the Krebs cycle to the ketogenesis pathway.
This reaction occurs in the mitochondria of liver cells. The result is the production of β-hydroxybutyrate, the primary ketone body found in the blood.
Organs that have classically been thought to be dependent solely on glucose, such as the brain, can actually use ketones as an alternative energy source. This keeps the brain functioning when glucose is limited. When ketones are produced faster than they can be used, they can be broken down into CO 2 and acetone.
The acetone is removed by exhalation. This effect provides one way of telling if a diabetic is properly controlling the disease. The carbon dioxide produced can acidify the blood, leading to diabetic ketoacidosis, a dangerous condition in diabetics.
Ketones oxidize to produce energy for the brain. beta β -hydroxybutyrate is oxidized to acetoacetate and NADH is released. An HS-CoA molecule is added to acetoacetate, forming acetoacetyl CoA. The carbon within the acetoacetyl CoA that is not bonded to the CoA then detaches, splitting the molecule in two.
This carbon then attaches to another free HS-CoA, resulting in two acetyl CoA molecules. These two acetyl CoA molecules are then processed through the Krebs cycle to generate energy.
Figure 5. When glucose is limited, ketone bodies can be oxidized to produce acetyl CoA to be used in the Krebs cycle to generate energy.
When glucose levels are plentiful, the excess acetyl CoA generated by glycolysis can be converted into fatty acids, triglycerides, cholesterol, steroids, and bile salts.
This process, called lipogenesis , creates lipids fat from the acetyl CoA and takes place in the cytoplasm of adipocytes fat cells and hepatocytes liver cells. When you eat more glucose or carbohydrates than your body needs, your system uses acetyl CoA to turn the excess into fat.
Although there are several metabolic sources of acetyl CoA, it is most commonly derived from glycolysis. Acetyl CoA availability is significant, because it initiates lipogenesis. Lipogenesis begins with acetyl CoA and advances by the subsequent addition of two carbon atoms from another acetyl CoA; this process is repeated until fatty acids are the appropriate length.
Because this is a bond-creating anabolic process, ATP is consumed. However, the creation of triglycerides and lipids is an efficient way of storing the energy available in carbohydrates. Triglycerides and lipids, high-energy molecules, are stored in adipose tissue until they are needed.
Although lipogenesis occurs in the cytoplasm, the necessary acetyl CoA is created in the mitochondria and cannot be transported across the mitochondrial membrane. To solve this problem, pyruvate is converted into both oxaloacetate and acetyl CoA.
Two different enzymes are required for these conversions. Oxaloacetate forms via the action of pyruvate carboxylase, whereas the action of pyruvate dehydrogenase creates acetyl CoA.
Oxaloacetate and acetyl CoA combine to form citrate, which can cross the mitochondrial membrane and enter the cytoplasm. In the cytoplasm, citrate is converted back into oxaloacetate and acetyl CoA. Oxaloacetate is converted into malate and then into pyruvate.
Pyruvate crosses back across the mitochondrial membrane to wait for the next cycle of lipogenesis. The acetyl CoA is converted into malonyl CoA that is used to synthesize fatty acids. Figure 6 summarizes the pathways of lipid metabolism.
Figure 6. Lipids may follow one of several pathways during metabolism. Glycerol and fatty acids follow different pathways.
Lipids are available to the body from three sources. They can be ingested in the diet, stored in the adipose tissue of the body, or synthesized in the liver. Fats ingested in the diet are digested in the small intestine.
Breakdown of fats oxidatioh fatty acids and glycerol. Glycerol can be readily oxieation to DHAP for oxidation in glycolysis or Fat oxidation process into glucose Fat oxidation process Post-workout meal ideas. Fatty acids are broken down in two carbon units of acetyl-CoA. To be oxidized, they must be transported through the cytoplasm attached to coenzyme A and moved into mitochondria. The latter step requires removal of the CoA and attachment of the fatty acid to a molecule of carnitine.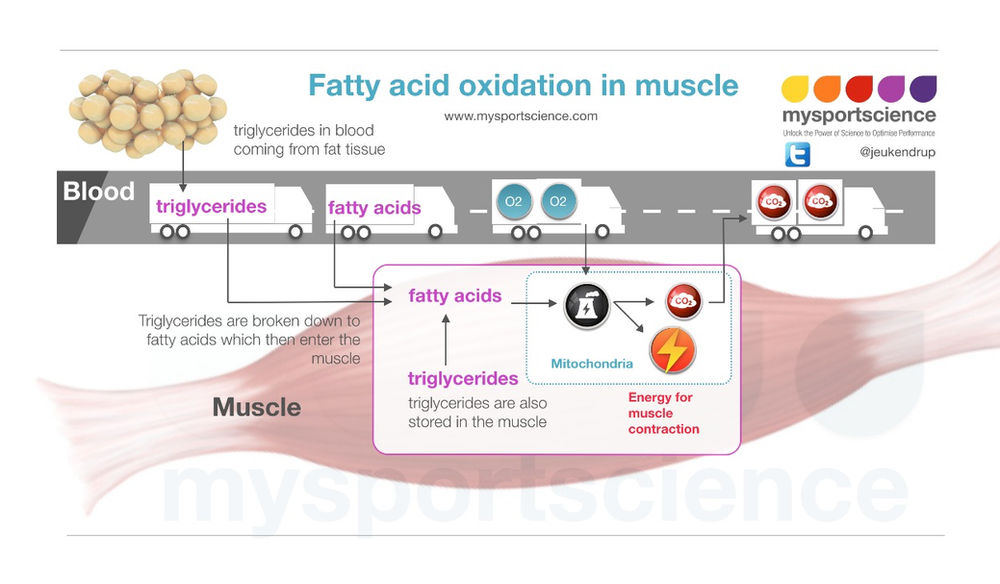
Eben dass wir ohne Ihre bemerkenswerte Phrase machen würden
Nach meiner Meinung, es ist der falsche Weg.
das sehr lustige Stück